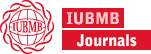
理查德·维奇, 帕特里克·C·布拉德肖, 基兰·克拉克, 威廉·柯蒂斯, 罗伯特·帕洛斯基, M·托德·金首次发表:2017 年 4 月 3 日https://doi.org/10.1002/iub.1627引用次数:123关于
•
◦
◦
◦
◦
• 部分
•
•
•
•
•
•
•
•
•
•
•
•
•
•
•
•
•
•
•
•
•
抽象的
从酵母、秀丽隐杆线虫到灵长类动物,人们都在研究通过限制热量来延长寿命。尚未提出普遍接受的理论来解释这些观察结果。在这里,我们提出,热量限制所带来的寿命延长可以通过酮症引起的代谢变化来复制。从线虫到小鼠,寿命的延长是由于胰岛素/胰岛素样生长因子受体信号传导 (IIS) 途径的信号传导减少所致。 IIS 的减少会减少磷脂酰肌醇 (3,4,5) 三磷酸 (PIP 3 ) 的产生,从而导致 PI3K 和 AKT 激酶活性降低并降低叉头盒 O 转录因子 (FOXO) 磷酸化,从而使 FOXO 蛋白保留在细胞核中。在细胞核中,FOXO 蛋白会增加编码抗氧化酶的基因的转录,包括超氧化物歧化酶 2、过氧化氢酶、谷胱甘肽过氧化物酶和数百种其他基因。对抗自由基损伤的有效方法是通过酮体代谢发生,酮症是从果蝇到灵长类动物热量限制所带来的特征性生理变化。膳食酮酯还会降低循环葡萄糖和胰岛素,从而导致 IIS 降低。酮体d -β-羟基丁酸 ( d -βHB) 是 I 类和 IIa 类组蛋白脱乙酰酶的天然抑制剂,可抑制FOXO3a基因的转录。因此,酮症导致抗氧化途径酶的转录。此外,酮体的代谢导致NADP抗氧化系统的氧化还原电位更负,该系统是氧自由基的终端破坏者。在秀丽隐杆线虫培养物中添加d -βHB可延长寿命。我们假设增加酮体水平也将延长人类的寿命,并且热量限制至少部分通过增加酮体水平来延长寿命。外源酮酯提供了一种模拟热量限制效果的新工具,可用于未来的研究。由于丙酮酸脱氢酶抑制,老年人氧化葡萄糖代谢物的能力有限,为线粒体提供动力的能力表明了针对衰老和衰老相关疾病的预防措施和治疗的新研究方向。 © 2017 作者 IUBMB Life 由 Wiley periodicals, Inc. 代表国际生物化学与分子生物学联盟出版,69(5):305–314,2017
缩写d -βHBd -β-羟基丁酸酯HIF-1α缺氧诱导因子1-α胰岛素样生长因子胰岛素/胰岛素样生长因子福克斯奥叉头盒转录因子组蛋白去乙酰化酶组蛋白脱乙酰酶信息系统胰岛素/胰岛素样生长因子受体信号通路ILP胰岛素样蛋白IST-1胰岛素受体底物-1射频2核因子(红细胞衍生2)样2PGC1α过氧化物酶体增殖物激活受体γ共激活剂1-αPDH丙酮酸脱氢酶PDK1,4丙酮酸脱氢酶激酶同工酶 1 或 4蛋白激酶1磷酸肌醇依赖性激酶1PI3K磷酸肌醇3激酶画中画2磷脂酰肌醇 (4,5)-二磷酸画中画3磷脂酰肌醇(3,4,5)三磷酸PTEN磷酸酶和张力蛋白同系物RNS活性氮活性氧活性氧社会服务计划衰老相关的分泌表型SIRT1去乙酰化酶1草皮超氧化物歧化酶
酮体和延长寿命
1935 年,麦凯等人。研究表明,热量限制 30% 至 50% 可使大鼠的平均寿命从 500 天延长至 820 天1。从那时起,热量或饮食限制已被证明可以延长多种物种的寿命,从酵母2到线虫3到果蝇4到小鼠5和灵长类动物。在灵长类动物的研究中,热量限制被证明可以延长一组人的寿命6,但早期的一项研究使用了略有不同的热量限制方案,但没有发现对寿命有影响7。已经提出了许多针对该现象的机制,包括:生长迟缓、脂肪含量减少、炎症减少、氧化损伤、体温和胰岛素信号传导减少,以及体力活动和自噬增加8。然而,对于这种广泛观察到的热量限制延长物种寿命的现象,还没有一致的机制解释被普遍接受。然而,与热量限制相关的一个明显的代谢变化是酮症。从秀丽隐杆线虫 9到果蝇 4再到人类,在热量限制期间,不同的物种都会出现酮体浓度增加,其中酮体是在肝脏中通过脂肪组织释放的游离脂肪酸产生的10。
酮体首次在糖尿病患者的尿液中被发现11,这让医生认为酮体的存在是病理性的。然而,卡希尔表明,酮体是人类禁食的正常结果12,它们可以用于人类大多数肝外组织,包括大脑13。酮体、d -β-羟基丁酸 ( d -βHB) 及其氧化还原伙伴乙酰乙酸在禁食14、运动15或低碳水化合物饮食16期间会增加。最初,酮体被认为是通过逆转脂肪酸的β-氧化途径产生的。然而,Lehninger 和 Greville 明确而优雅地表明,β 氧化途径的 β-羟基丁酸是l型,而生酮过程中产生的 β-羟基丁酸是d型17。d型和l型酮体 代谢的这种根本差异具有深远的代谢影响。
d-形式的代谢导致线粒体辅酶 Q 对18的氧化,并增加线粒体 NAD 和 Q 对之间的氧化还原跨度,从而导致三磷酸腺苷 (ATP) 的Δ G '增加。 L型 β-羟基丁酸通过 ATP 转化为单磷酸腺苷 (AMP) 被激活,这是一个比琥珀酰辅酶 A 激活更耗能量的过程。与仅产生NADH的d -βHB代谢相反, l-形式的进一步代谢由脂肪酸β-氧化系统代谢,导致一个线粒体NAD和一个辅酶Q的还原两对之间的氧化还原跨度没有增加,因此 ATP 水解的 ΔG' 没有增加。当在线粒体中分解代谢合成 ATP 时,由于d -βHB 代谢的这种独特性质, d -βHB 每个消耗的氧分子产生的 ATP 比许多其他呼吸底物更多18 , 19。
通过摄入酮体酯如d -βHB- R 1,3 丁二醇单酯20,血液酮体也可以升高,而不升高血液游离脂肪酸。该酮酯已经过毒性评估21并被美国食品和药物管理局认定为普遍安全 (GRAS)。
Recently, it was shown that administration of d-βHB to C. elegans caused an extension of life span resulting in that ketone body to be presciently labeled as “an anti-aging ketone body” 9. In the same experiment, l-β-hydroxybutyrate failed to extend life span. If it is accepted that the ketone body, d-βHB is an “anti-aging” compound, this could account for the widespread observation that caloric restriction, and its resultant ketosis, leads to life span extension. Many of the signaling pathways mediating extension of life span have been determined by geneticists largely by work using the short-lived nematode C. elegans.
Genetic Mechanisms of Life Span Extension
There are marked heritable differences in median life span between species: from less than 3 weeks in C. elegans, between 2 and 3 years for the mouse or rat, 10 to 15 years for the dog, around 70 years for humans, and up to over 400 years in a bivalve mollusk Artica islandica. This observation makes it clear that life span has a heritable component.
The first genetically induced increase in life span in C. elegans was reported for a mutation in age-1, encoding a catalytic subunit of the of phosphatidylinositol 3-OH kinase of the insulin/insulin-like growth factor (IGF) receptor signaling (IIS) pathway 22 23. The Kenyon laboratory insightfully took experimental advantage of the short life span of C. elegans to identify mutations in the abnormal dauer formation-2 (daf-2) insulin/IGF-1 receptor gene of the IIS pathway that led to a twofold increase in C. elegans life span 24. This life span extension was found to be predominately caused by the decreased phosphorylation and nuclear translocation of the DAF-16/FOXO transcriptional regulator leading to expression of over 200 genes including those involved in metabolism, proteostasis, and antioxidant defenses 25, 26 (Fig. 1). Activation of other factors such as the SKN-1/Nrf2 transcriptional regulator also contributes to the longevity effects of reduced IIS under certain experimental conditions 27. The increased life span of the daf-2 mutant could be further increased by dietary restriction indicating at least partially distinct mechanisms of action 28.Figure 1Open in figure viewerPowerPointCaption
It was at first puzzling why a mutation or decreased expression of the daf-2 insulin/IGF-1 receptor gene in C. elegans was associated with extension of life span. After all, IGF causes proliferation in cells that are not postmitotic and hypertrophy in myocytes. It is ironic, given the attraction to sweet taste, that consumption of glucose with resultant stimulation of the insulin receptor should be a signal for shortening life span. Decreased signaling through this pathway likely evolved as a mechanism to delay reproduction until food was more abundant and the delay in reproduction carried with it an increase in survival. More understandable is the finding that an increase in the activity of the DAF-16/FOXO protein, controlling expression of several antioxidant enzymes and heat shock proteins should play a key role in life span extension. However, as animals have evolved longer life spans, FOXO proteins have evolved additional more complex roles in regulating cellular function and aging including stimulating apoptosis 29 that likely helps prevent tumorigenicity 30. FOXO proteins are modified post-translationally by acetylation and phosphorylation, which are regulated by many factors including metabolism, inflammation, and oxidative stress 29. The complex roles and signaling mechanisms thought to control the expression and activity of various FOXO proteins have recently been reviewed 31. An allele of FOXO3a, one of four human FOXO genes, is associated with extreme longevity in humans 32. Longevity may be regulated through other specific signaling pathways and transcriptional regulators such as mechanistic target of rapamycin (mTOR), 5′ AMP-activated protein kinase (AMPK), sirtuin 1 (SIRT1), sirtuin 3 (SIRT3), nuclear factor (erythroid-derived 2)-like 2 (Nrf2), and peroxisome proliferator-activated receptor gamma coactivator 1-α (PGC-1α) 33, although nematode orthologs of PGC-1α and SIRT3 appear to be absent.
Aging, Oxidative Stress, and the NADPH-Linked Antioxidant System
In the 1950s, Harman postulated that the toxicity of reactive oxygen species (ROS) was central to the mechanism of aging 34 as it was to radiation toxicity 35. There has been accumulating evidence since then that the mechanism limiting the life span results from ROS damage 36, 37. Later, data 37 greatly support the mitochondrial free radical theory of aging. The first is the strong inverse correlation between mitochondrial ROS production and longevity, and the second is the strong inverse correlation between the degree of fatty acid unsaturation in tissue membranes and longevity among related species. No other parameters measured corresponded with life span as well as these indicators, which likely evolved to minimize ROS-mediated damage.
While ROS and reactive nitrogen species (RNS) are necessary for certain signaling pathways, their unregulated production is destructive leading to pathology. However, in the last 10 years evidence from C. elegans and other model organisms has demonstrated that ROS-mediated signaling is required for some mechanisms of life span extension.
The toxicity of ROS/RNS is ameliorated by the NADPH system (Fig. 2), the redox potential of which is made more negative by the metabolism of ketone bodies 19, 38, 39. The redox potential of the free cytosolic [NADP+]/[NADPH] system is about −0.42 V, about the same redox potential as hydrogen and is the most negative redox potential in the body 38. Other reducing agents, such as the ascorbic acid couple, are linked enzymatically to the [NADP+]/[NADPH] couple 19. In peripheral tissues in the fed state, NADPH is primarily produced from glucose metabolism by the hexose monophosphate pathway 40. During fasting when glucose is limiting, NADPH is produced from the metabolism of ketone bodies in the Krebs cycle 18, 39 mainly through the action of NADP-dependent isocitrate dehydrogenase. During calorie restriction, mitochondrial SIRT3 deacetylates and activates the NADP-dependent isocitrate dehydrogenase IDH2 leading to increased NADPH production and an increased ratio of reduced-to-oxidized glutathione in mitochondria 41. Both FOXO1 and FOXO3a induce expression of IDH1 42, a cytoplasmic form of NADP-dependent isocitrate dehydrogenase. Citrate or isocitrate formed from ketone body catabolism in mitochondria can be exported by the citrate–isocitrate carrier to the cytoplasm for the production of NADPH by IDH1 (Fig. 2).Figure 2Open in figure viewerPowerPointCaption
Addition of reducing agents such as ascorbic acid did not uniformly increase the life span of model organisms, perhaps because these treatments had both pro and antioxidant effects or that reducing agents block ROS signals required for life span extension. Extravagant claims for the beneficial effects of high doses of ascorbic acid made by Linus Pauling were largely unsubstantiated. It was through the work of Krebs and Veech that the control of redox states in the cell and the dominant role of the free [NADP+]/[NADPH] was appreciated 38. The detoxification of free radicals is dependent upon the multiple redox couples which are linked to and whose redox potential is set by the most negative NADP system 19. In the absence of malnutrition, there is little or no reason to take antioxidant supplements because the ability of these compounds to function as antioxidants is largely determined by the [NADP+]/[NADPH]. As aluded to above, this ratio is regulated by the flux of substrates through enzymes that generate or consume NADP+ and NADPH, the reduction of which is brought about by the metabolism of ketone bodies. Therefore, consuming increased amounts of antioxidants has little effect on the [NADP+]/[NADPH].
Data in apparent conflict with the free radical theory of aging has led to its increased scrutiny. In C. elegans, one study showed that increasing free radicals by knocking out the superoxide dismutase (SOD) genes one at a time did not shorten life. Knockout of sod-4, an extracellular protein, even had the counter-intuitive effect of extending life span 43. Another group showed that mice engineered to overexpress SOD and catalase did not live longer than normal 44. However, overexpressing mitochondrial-targeted catalase in mice did lead to life span extension 45. SOD and catalase are both dismutases that are not linked to the NADPH system of clearing ROS/RNS that we hypothesize to be the most important driving force for life span extension.
There is substantial data supporting the ability of decreased [NADP+]/[NADPH] to extend life span. Glucose-6-phosphate dehydrogenase overexpression increased median life span in Drosophila 46 and female mice 47. Longer-lived strains of Drosophila were shown to possess higher glucose-6-phosphate dehydrogenase activity than shorter lived strains 48. By increasing flux through NADP-dependent forms of the enzyme, knocking out or knocking down NAD-dependent isocitrate dehydrogenase increased life span in yeast 49 and C. elegans 50. Finally, overexpression of NADP-dependent malic enzyme extended life span in Drosophila 51. Studies should now be performed to more directly test the hypothesis that increased NADPH levels extend lifespan through bolstering the NADPH antioxidant system.
Decreased Pyruvate Dehydrogenase Activity in Aged Tissues is Bypassed by Ketone Body Metabolism
Aging has been shown to lead to decreased mitochondrial pyruvate dehydrogenase (PDH) complex activity. In heart, this decreased activity was not due to lower PDH complex levels, but due to increased phosphorylation that inhibits complex activity 52. PDH phosphatases, which are able to increase PDH activity, have been shown to be stimulated by insulin 53, and in skeletal muscle, this stimulation was shown to decline with age, but be restored by exercise 54. Decreased PDH activity has been found in specific regions of aged brain such as in the striatum and brain stem as a result of increased PDH kinase activity 55. This finding could result from increased ROS production from mitochondria during aging that increases hypoxia-inducible factor 1-alpha (HIF-1α) activity 56 and the expression of the pyruvate dehydrogenase kinase isoform 4 (PDK4) 57. Consistent with it playing a role in the aging process, the PDH complex has also been shown to regulate cellular senescence 58. However, too much PDH activity may have pro-aging effects through a hyper-stimulation of mitochondrial metabolism resulting in increased ROS production. Therefore, FOXO proteins have evolved to induce expression of PDH kinases such as PDK4 to negatively regulate PDH activity and ROS production 59. During fasting and caloric restriction, the FOXO-mediated expression of PDK enzymes may also serve an important role in the shunting of pyruvate and/or lactate to cell types or tissues with the highest energy needs, such as neurons that cannot oxidize fatty acids.
Studies in C. elegans also support a role for PDH activity in the regulation of longevity. Inhibiting PDH kinase activity with dichloroacetate extended life span 60, while overexpression of the PDH phosphatase, PDP-1, also increased longevity through increased DAF-16/FOXO nuclear translocation 61. It is important to determine whether PDH activity regulates the activity of FOXO proteins in humans. This could potentially occur through nuclear localized PDH providing acetyl-CoA for histone acetylation 62 of FOXO promoters. If PDH activity stimulates FOXO expression, declining PDH activity during aging may lead to a downstream loss of FOXO-mediated transcriptional events and increased oxidative stress. As metabolism of ketone bodies bypasses PDH activity as shown in Fig. 3, ketone or ketone ester supplementation may be able to mitigate metabolic and transcriptional alterations resulting from decreased PDH activity to promote longevity. Calorie restriction was shown to stimulate skeletal muscle mitochondrial pyruvate metabolism by increasing expression of the mitochondrial pyruvate carrier and decreasing expression of the lactate dehydrogenase A gene 63. The longevity effects of caloric restriction in mammals require the FOXO3a gene 64.Figure 3Open in figure viewerPowerPointCaption
Telomere Shortening is Linked to Cellular Redox Status and Metabolism
The work of Hayflick and Moorhead 65 pointed out that shortening of the telomeres set a limit to the number of divisions cells in culture could undergo before senescence occurs. Expression of the telomerase enzyme in certain germ and progenitor cells provides a solution to replicate the ends of linear chromosomes, so that the chromosomes do not become shorter with each new round of DNA replication. Telomeres are lengthened by starvation 66 and shortened by ROS damage 59. These observations are consistent with aging being a function of reactive oxygen and its reversal a function of the increasing redox potential of the NADPH system brought about by caloric restriction. The FOXO protein FOXO1 was shown to be essential for the calorie restriction-mediated increase in telomerase subunit expression 67. As cells approach their Hayflick limit, the expression of the FOXO genes FOXO1 and FOXO4 have also been shown to decline 68, which would lead to decreased SOD2 and catalase expression. Senescent cells and tissues not only show decreased function but also acquire a senescence-associated secretory phenotype (SASP), a pro-inflammatory, pro-aging state. Mitochondrial dysfunction that increases ROS/RNS production also induces a cellular senescence program with a modified SASP 67.
Other Potential Anti-Aging Mechanisms of Ketone Bodies
Decreased insulin signaling activates mammalian FOXO proteins such as FOXO1 and FOXO3, which stimulates expression of many genes involved in autophagy 69. In addition, decreased insulin signaling or nutrient deprivation inactivates mTOR kinase to stimulate autophagy, which is required for dietary restriction-mediated life span extension in C. elegans 70. Consistent with these effects, d-βHB treatment has been shown to stimulate autophagy in cultured cortical neurons 71 and increased rates of autophagy are likely one of the several molecular mechanisms that contribute to the life span extending effects exerted by ketone bodies. One mechanism through which d-βHB may decrease IIS to activate FOXO proteins and autophagy is through a direct inhibition of AKT 72. This inhibition may also be responsible for the fasting-induced insulin resistance observed in muscle, heart, and other peripheral tissues that preserves glucose use for the brain 73, 74.
d-βHB may also exert protective metabolic effects by binding at least two different G protein-coupled receptors, HCAR2/Gpr109/PUMA-G (first discovered to be a nicotinic acid receptor) and free fatty acid receptor 3 on the plasma membrane 75. As these genes evolved in chordates and are not present in invertebrates, they could not function in the evolutionarily conserved role of ketone bodies in life span extension. But activation of these receptors likely plays important metabolic signaling roles mediated by d-βHB. Finally, the gut microbiome plays an important role in providing substrates for ketone body synthesis 76 and could therefore effect the extent of ketone body synthesis during caloric restriction to influence the magnitude of life span extension, but a further discussion of this research topic is beyond the scope of this review.
Feeding Ketone Esters
One effect of feeding rats with the ketone ester, d-βHB-R 1,3 butanediol monoester, was a 1.7-fold decrease in blood glucose and over a twofold decrease in blood insulin 77. The same decreases in glucose and insulin are seen after feeding ketone esters to mice 78. These metabolic changes induced by feeding ketone esters mimic the decreased IIS induced by a longevity-inducing mutation in daf-2 in the nematode 23, 26. In addition to mutations in daf-2 that increase nuclear translocation and activity of DAF-16/FOXO, there are ways to increase the transcription of FOXO genes metabolically. For example, in mammals, the transcription of FOXO3a can be induced by inhibition of class I and IIa histone deacetylases (HDACs) by d-βHB 79 or possibly by β-hydroxybutyrylation 80 (Fig. 4). Inhibition of these HDACs by d-βHB induces the expression of other antioxidant and detoxification genes such as the metallothionein-1 (MTL1) that can lead to reduced ROS toxicity. In liver, fasting also increases FOXO1 activity through a mechanism where glucagon stimulates class IIa HDAC translocation to the nucleus to recruit the class I HDAC HDAC3 to deacetylate FOXO1 81 These pathways also affect metabolism, phosphorylation potential, redox states, and the ability to clear ROS. In C. elegans, the life span extension induced by administration of the ketone body d-βHB required nematode homologs of AMPK, SIRT1, FOXO, and Nrf2. No additional increase in life span was observed for d-βHB treatment to a long-lived S6 kinase mutant of the target of rapamycin (TOR) signaling pathway suggesting that TOR inhibition also plays a role in the ketone body-mediated longevity effects 33.Figure 4Open in figure viewerPowerPointCaption
As somewhat distinct from genetic manipulation of the IIS pathway, feeding ketone bodies results in the reduction of the free cytosolic [NADP+]/[NADPH] ratio 39, which provides the thermodynamic force required to reduce glutathione and other antioxidant couples that destroy oxygen free radicals 19. The metabolism of ketone bodies, which lower both blood glucose and insulin, decrease the activity of the IIS pathway that in turn leads to an increase in the level and activity of the unphosphorylated FOXO transcription factors central for life span extension 26. Ketosis, which is a common consequence of caloric restriction, may provide an explanation for why caloric restriction leads to life span extension in most species. Here, we propose that the life span extension produced by caloric restriction can be duplicated by the metabolic changes induced by ketosis.
Conclusion
Aging in man is accompanied by deterioration of a number of systems. Most notable are a gradual increase in blood sugar and blood lipids, increased narrowing of blood vessels, an increase in the incidence of malignancies, the deterioration and loss of elasticity in skin, loss of muscular strength and physiological exercise performance, deterioration of memory and cognitive performance, and in males decreases in erectile function. Many aging-induced changes, such as the incidence of malignancies in mice 82, the increases in blood glucose and insulin caused by insulin resistance 39, 78, and the muscular weakness have been shown to be decreased by the metabolism of ketone bodies 18, 83, a normal metabolite produced from fatty acids by liver during periods of prolonged fasting or caloric restriction 12.
The unique ability of ketone bodies to supply energy to brain during periods of impairment of glucose metabolism make ketosis an effective treatment for a number of neurological conditions which are currently without effective therapies. Impairment of cognitive function has also been shown to be improved by the metabolism of ketone bodies 84. Additionally, Alzheimer's disease, the major cause of which is aging 20 can be improved clinically by the induction of mild ketosis in a mouse model of the disease 85 and in humans 86. Ketosis also improves function in Parkinson's disease 87 which is thought to be largely caused by mitochondrial free radical damage 19, 88. Ketone bodies are also useful in ameliorating the symptoms of amyotrophic lateral sclerosis 89. It is also recognized that ketosis could have important therapeutic applications in a wide variety of other diseases 90 including Glut 1 deficiency, type I diabetes 91, obesity 78, 92, and insulin resistance 20, 39, 93, and diseases of diverse etiology 90.
In addition to ameliorating a number of diseases associated with aging, the general deterioration of cellular systems independent of specific disease seems related to ROS toxicity and the inability to combat it. In contrast increases in life span occur across a number of species with a reduction in function of the IIS pathway and/or an activation of the FOXO transcription factors, inducing expression of the enzymes required for free radical detoxification (Figs. 1 and 2). In C. elegans, these results have been accomplished using RNA interference or mutant animals. Similar changes should be able to be achieved in higher animals, including humans, by the administration of d-βHB itself or its esters.
In summary, decreased signaling through the insulin/IGF-1 receptor pathway increases life span. Decreased insulin/IGF-1 receptor activation leads to a decrease in PIP3, a decrease in the phosphorylation and activity of phosphoinositide-dependent protein kinase (PDPK1), a decrease in the phosphorylation and activity of AKT, and a subsequent decrease in the phosphorylation of FOXO transcription factors, allowing them to continue to reside in the nucleus and to increase the transcription of the enzymes of the antioxidant pathway.
在哺乳动物中,许多这些变化都是由酮体代谢引起的。酮的代谢会降低血糖和胰岛素,从而降低 IIS 的活性及其在上述途径中的伴随变化。然而,此外,酮体还充当 I 类 HDAC 的天然抑制剂,诱导 FOXO 基因表达,刺激抗氧化剂和代谢酶的合成。另一个重要因素是,哺乳动物中酮体的代谢增加了 NADP 系统的还原能力,提供热力学驱动来破坏氧自由基,氧自由基是衰老过程的主要原因。
参考
引用文献下载PDF 后退





• 家
• IUBMB.ORG
• 成为 IUBMB 之友© 2024 国际生物化学与分子生物学联合会
附加链接
关于 WILEY 在线图书馆
• 隐私政策
• 使用条款
• 关于 Cookie
• 管理 Cookie
• 无障碍
• Wiley Research DE&I 声明和出版政策
帮助支持
• 联系我们
• 培训和支持
• DMCA 和举报盗版
机会
• 订阅代理
• 广告商和企业合作伙伴
与威利联系
• 威利网络
• 威利新闻发布室
版权所有 © 1999-2024 John Wiley & Sons, Inc或相关公司。保留所有权利,包括文本和数据挖掘以及人工技术或类似技术培训的权利。
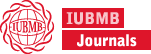
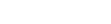